The discovery of a new pathway that underpins MRSA’s (Methicillin-resistant Staphylococcus aureus) extreme resistance to antibiotics has shed light on how this formidable superbug outlasts potent drugs. This insight could pave the way for innovative approaches to combat antibiotic-resistant bacteria, a pressing public health threat affecting millions worldwide.
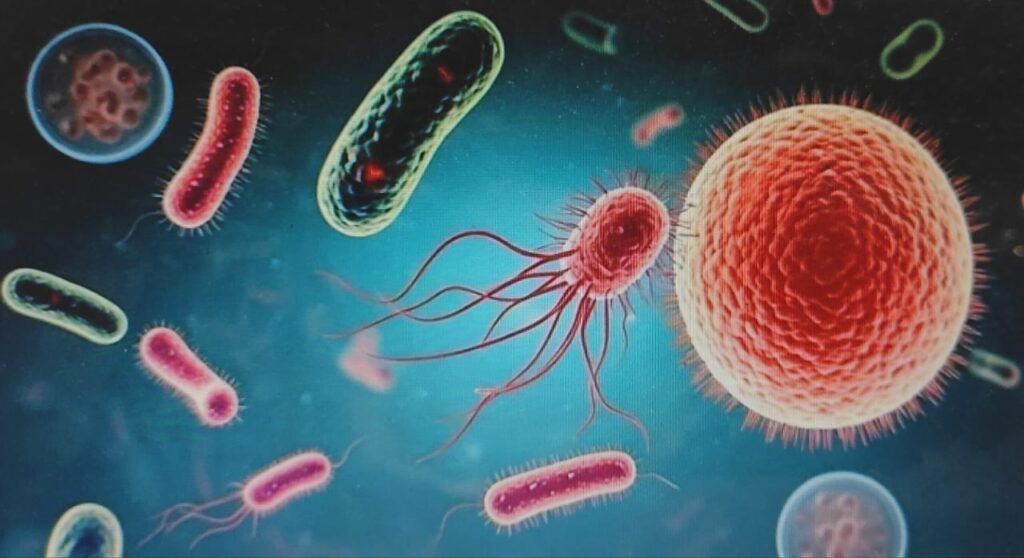
The Impact of Antibiotic Resistance on Modern Medicine
Antibiotics have been instrumental in transforming modern medicine, significantly lowering mortality rates associated with infectious diseases. However, the effectiveness of antibiotics has waned with the rise of resistant bacteria. This resistance has largely stemmed from overuse and misuse of antibiotics, allowing certain bacteria to evolve and thrive in antibiotic-rich environments. These strains, often referred to as “superbugs,” are capable of resisting multiple classes of antibiotics, rendering conventional treatments ineffective.
One of the most notorious superbugs, MRSA, presents a formidable threat to human health, especially in hospitals where vulnerable patients are at a heightened risk of infection. MRSA strains have developed mechanisms to survive even the strongest antibiotics, making them a focal point of research aimed at understanding and curbing antibiotic resistance.
How MRSA Became Resistant to Antibiotics
Initially, Staphylococcus aureus infections were treated effectively with β-lactam antibiotics. These drugs work by inhibiting bacterial cell wall synthesis, thus halting bacterial growth and division. However, over time, S. aureus evolved resistance mechanisms, primarily through the acquisition of the mecA gene, which codes for a unique protein known as Penicillin Binding Protein 2a (PBP2a). This protein’s structure allows it to evade the effects of β-lactam antibiotics, preserving the bacteria’s ability to grow even in the presence of these drugs.
Methicillin, a penicillin-derived antibiotic, was introduced to counteract these resistant strains. Unfortunately, MRSA strains quickly adapted, compromising methicillin’s efficacy. Current MRSA infection mortality rates can range from 15% to 60%, highlighting the urgent need for new therapeutic strategies.
Investigating MRSA’s Mechanisms of Methicillin Resistance
To uncover how MRSA withstands methicillin, researchers examined multiple strains of S. aureus with varying levels of resistance. This study utilized wild-type strains (non-resistant), low-resistance MRSA, and highly resistant clinical MRSA strains. Through exposure to different methicillin concentrations, researchers tracked structural and genetic changes in the bacteria to understand how they adapted and survived.
The team employed high-resolution atomic force microscopy (AFM) to observe changes in the bacterial cell wall’s peptidoglycan structure, a critical component that methicillin targets to inhibit bacterial growth. By studying the structural responses of MRSA to increasing methicillin doses, scientists gained insight into the specific mutations and mechanisms MRSA uses to circumvent methicillin’s inhibitory effects.
Key Findings: The Role of Genetic Mutations in MRSA’s Antibiotic Resistance
The study identified a two-step process through which MRSA evolves from methicillin-susceptible to highly resistant.
- Acquisition of the mecA Gene: This initial step allows the production of PBP2a, which bypasses the regular function of native PBP2 (another penicillin-binding protein). As a result, MRSA can continue its cellular processes despite the presence of methicillin, which would typically disrupt cell wall synthesis.
- Development of Potentiator Mutations: The researchers discovered specific mutations in the rpoB gene (coding for an RNA polymerase subunit essential for bacterial DNA replication) that significantly enhance MRSA’s resistance. These mutations eliminate MRSA’s dependency on PBP1 for cell division, effectively creating an alternative pathway that bypasses methicillin’s mode of action.
These genetic alterations not only bolster MRSA’s resistance to methicillin but also allow the bacteria to thrive in higher antibiotic concentrations that would typically kill non-resistant strains. The mutations in the rpoB gene, along with additional potentiator mutations in other genes (such as rpoC, rel, clpXP, and gdpP), collectively reinforce MRSA’s resistance by reshaping its cell division mechanisms.
How Potentiator Mutations Reinforce MRSA Resistance
Potentiator mutations identified in this study play a crucial role in MRSA’s high-level resistance. These mutations contribute to a novel resistance pathway by altering the bacterial cellular processes in a way that bypasses the conventional pathways affected by β-lactam antibiotics like methicillin.
PBP2a, the protein produced due to the mecA gene, exhibits poor binding to methicillin and other β-lactam antibiotics. While PBP2a alone cannot entirely replace the functions of native PBPs (penicillin-binding proteins), it can form complexes with them, enhancing their activity under antibiotic stress. This dimerization fortifies MRSA’s defenses, as these complexes become less susceptible to methicillin’s actions.
Bypassing Methicillin’s Mode of Action
The study revealed that MRSA strains with potentiator mutations operate through an alternative cell division pathway independent of PBP1 activity. This pathway, activated by potentiator mutations, renders MRSA less reliant on PBP1, thus evading methicillin’s inhibitory effects on cell wall synthesis.
The findings also suggest that only a small percentage of MRSA cells in a population may initially acquire these potentiator mutations. However, when exposed to high methicillin levels, these mutation-carrying cells survive while others die off. Over time, these mutated strains dominate the bacterial population, leading to highly resistant MRSA strains that persist in both healthcare and community settings.
Implications of the Findings on MRSA Research and Treatment
This study highlights the complexity of MRSA’s resistance mechanisms and underscores the role of genetic mutations in antibiotic resistance. Previously, only auxiliary genes associated with low-level resistance were recognized in MRSA strains. However, this new pathway involving potentiator mutations expands the understanding of high-level resistance, presenting a more intricate genetic foundation for MRSA’s resilience against methicillin.
The implications for treatment are significant. Traditional approaches may no longer suffice, as MRSA’s resistance now involves sophisticated genetic adaptations that allow it to bypass the inhibitory actions of methicillin altogether. By targeting these potentiator mutations and the unique pathways they create, future treatments may disrupt MRSA’s survival mechanisms, potentially reducing its spread and impact.